The Genomics Education Partnership: Successful Integration of Research Into Laboratory Classes at a Diverse Group of Undergraduate Institutions
Genomics is not only essential for students to understand biology but also provides unprecedented opportunities for undergraduate research. The goal of the Genomics Education Partnership (GEP), a collaboration between a growing number of colleges and universities around the country and the Department of Biology and Genome Center of Washington University in St. Louis, is to provide such research opportunities. Using a versatile curriculum that has been adapted to many different class settings, GEP undergraduates undertake projects to bring draft-quality genomic sequence up to high quality and/or participate in the annotation of these sequences. GEP undergraduates have improved more than 2 million bases of draft genomic sequence from several species of Drosophila and have produced hundreds of gene models using evidence-based manual annotation. Students appreciate their ability to make a contribution to ongoing research, and report increased independence and a more active learning approach after participation in GEP projects. They show knowledge gains on pre- and postcourse quizzes about genes and genomes and in bioinformatic analysis. Participating faculty also report professional gains, increased access to genomics-related technology, and an overall positive experience. We have found that using a genomics research project as the core of a laboratory course is rewarding for both faculty and students.
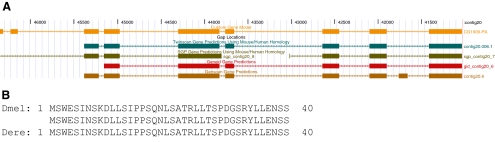
Shaffer CD, Alvarez C, Bailey C, et al. The Genomics Education Partnership: Successful Integration of Research Into Laboratory Classes at a Diverse Group of Undergraduate Institutions. CBE Life Sci Educ. 2010;9(1):55‐69. doi:10.1187/09-11-0087